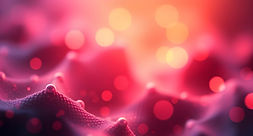
Fullerene Applications
Fullerene: Background, Key Concepts, Trends, Challenges, and Future Prospects
1. Introduction
Fullerenes are a fascinating class of carbon allotropes composed entirely of carbon atoms, where the atoms are arranged in a hollow, spherical, cylindrical, or other polyhedral structures. The discovery of fullerenes in the mid-1980s revolutionized the field of nanotechnology and materials science. These molecules have unique properties that distinguish them from other forms of carbon, such as graphite and diamond, which have paved the way for innovations in a wide range of applications, including electronics, medicine, energy storage, and materials science. This paper will explore the background of fullerenes, the key concepts surrounding their structure and properties, the current trends in fullerene research, the challenges faced in their synthesis and applications, and the future prospects for fullerene-based technologies.
2. Background Information
2.1 Discovery of Fullerenes
The discovery of fullerenes can be traced back to 1985, when scientists Harold Kroto, Richard Smalley, and Robert Curl at Rice University identified the first fullerene molecule, C60, which is a spherical molecule consisting of 60 carbon atoms. This molecule, often referred to as a “buckyball” due to its resemblance to a soccer ball, was named after architect Buckminster Fuller, whose geodesic domes resemble the structure of the molecule. The breakthrough discovery of C60 was followed by the identification of other fullerene molecules, including C70, C76, and C84, which are structurally similar but differ in the number of carbon atoms.
Fullerenes represent the third allotrope of carbon, after graphite and diamond. Unlike graphite, which has a planar structure of carbon atoms bonded in hexagonal rings, or diamond, which has a tetrahedral structure, fullerenes have a spherical or cylindrical shape, with carbon atoms bonded in pentagonal and hexagonal arrangements.
2.2 Fullerene Types
Fullerenes can be classified into several categories:
• Spherical Fullerenes: The most well-known example is C60, which forms a truncated icosahedron. Other variations, such as C70, C76, and C84, exhibit similar spherical structures with different numbers of carbon atoms.
• Cylindrical Fullerenes (Nanotubes): Carbon nanotubes (CNTs) are long, cylindrical fullerenes that exhibit remarkable mechanical strength and electrical conductivity. CNTs are further divided into single-walled (SWNTs) and multi-walled (MWNTs) nanotubes, depending on the number of concentric graphene layers.
• Polyhedral Fullerenes: These fullerenes feature more complex, polyhedral shapes, and can include a variety of different carbon atom arrangements.
3. Key Concepts and Properties of Fullerenes
3.1 Molecular Structure and Bonding
Fullerenes are characterized by a unique arrangement of carbon atoms in a combination of pentagons and hexagons. In the case of C60, the structure consists of 12 pentagons and 20 hexagons, forming a highly symmetrical shape. The carbon-carbon bonds in fullerenes are a mix of single and double bonds, with the structure exhibiting both sp2 hybridization (as seen in graphite) and a curvature that distinguishes fullerenes from other carbon allotropes.
3.2 Physical and Chemical Properties
Fullerenes possess several distinctive properties that make them suitable for various applications:
• Stability: Fullerenes are highly stable due to their closed, spherical structure, which distributes strain uniformly across the molecule.
• Electrical Conductivity: Fullerenes exhibit interesting electronic properties, including semiconducting behavior, making them useful in electronics and optoelectronics.
• Reactivity: The unique structure of fullerenes allows for a range of chemical modifications. Fullerenes can undergo addition reactions, such as the formation of fullerene derivatives by reacting with other molecules like hydrogen, metals, or functional groups.
• Solubility: Fullerenes are generally insoluble in water but can dissolve in organic solvents such as toluene, chloroform, and benzene, which aids in their processing and applications.
3.3 Fullerene Derivatives
Fullerenes can be chemically modified to enhance their properties for specific applications. This can include the attachment of functional groups to the carbon atoms, allowing for better solubility, reactivity, and even targeting specific biological cells. Fullerene derivatives are widely studied in drug delivery systems, where they can be used to transport drugs to specific cells or tissues.
4. Current Trends in Fullerene Research
4.1 Fullerene-Based Nanotechnology
The potential of fullerenes in nanotechnology has driven much research into their applications. Fullerenes and their derivatives are being explored for use in a variety of nanodevices, such as molecular sensors, molecular motors, and drug delivery systems. Carbon nanotubes, a type of fullerene, are particularly exciting due to their exceptional mechanical strength, electrical conductivity, and potential for use in flexible electronics.
4.2 Energy Storage and Conversion
Fullerenes and their derivatives are also being studied for their potential in energy storage and conversion systems. Their high surface area and ability to accept and donate electrons make them suitable for use in supercapacitors, lithium-ion batteries, and other energy storage devices. Additionally, fullerenes have shown promise in solar cells, particularly in organic photovoltaics, where they are used as electron acceptors in the photoelectric conversion process.
4.3 Biomedical Applications
The biomedical applications of fullerenes are vast and include their use as antioxidants, drug delivery agents, and in cancer therapy. Fullerenes can act as free radical scavengers, and studies have shown that they can be used to protect cells from oxidative damage. Moreover, functionalized fullerenes are being explored as carriers for delivering drugs, genes, and other therapeutic agents to specific cells or tissues. The potential use of fullerenes in photodynamic therapy for cancer treatment is also under investigation.
4.4 Environmental Remediation
Fullerenes have shown potential in environmental applications, including water purification, soil remediation, and carbon capture. Fullerenes and their derivatives can adsorb various pollutants, and research is ongoing to explore their use in filtering heavy metals, pesticides, and other toxic substances from the environment.
5. Challenges in Fullerene Research and Applications
5.1 Synthesis and Cost
One of the primary challenges in fullerene research and commercialization is the difficulty and cost of synthesis. While fullerenes can be produced through arc discharge or laser ablation methods, these processes are energy-intensive and often result in low yields. New methods for synthesizing fullerenes more efficiently and at a lower cost are actively being researched.
5.2 Toxicity and Biocompatibility
Despite the promising biomedical applications, the toxicity and biocompatibility of fullerenes are concerns that must be addressed. Some studies have shown that fullerenes, particularly in their unmodified form, may cause toxicity in certain biological systems. Therefore, thorough toxicity testing and modification of fullerenes to improve their biocompatibility are essential for their safe use in medicine.
5.3 Environmental Impact
While fullerenes hold promise for environmental remediation, the potential impact of large-scale production and disposal of fullerenes is not yet fully understood. The long-term environmental effects of fullerene nanoparticles, particularly in aquatic and terrestrial ecosystems, require further investigation.
6. Future Prospects for Fullerene-Based Technologies
6.1 Advancements in Synthesis
Future research in fullerene synthesis will likely focus on developing more efficient and scalable methods. Advancements in green chemistry and more sustainable production techniques may reduce the environmental impact and cost of fullerene production. Moreover, breakthroughs in controlled synthesis could lead to new forms of fullerenes with tailored properties for specific applications.
6.2 Fullerene-Based Electronics and Materials
Fullerenes are expected to play a critical role in the development of next-generation electronic devices. Their unique electronic properties make them suitable for use in organic light-emitting diodes (OLEDs), transistors, and sensors. Additionally, fullerene-based composites are being explored for use in advanced materials, such as lightweight, high-strength polymers and coatings.
6.3 Expanding Biomedical Applications
The use of fullerenes in biomedicine will continue to expand as researchers develop more functionalized derivatives that are biocompatible and capable of targeting specific disease cells. In particular, their role in cancer therapy, drug delivery, and regenerative medicine holds significant promise.
6.4 Environmental and Energy Applications
Fullerenes will likely play an increasingly important role in environmental and energy applications, particularly in energy storage and solar energy conversion. The ongoing development of fullerene-based materials for batteries, supercapacitors, and solar cells could help drive the transition to more sustainable energy systems.
7. Conclusion
Fullerenes represent a class of materials with extraordinary properties that have captivated researchers and industry alike. Their unique molecular structure opens up a wide range of possibilities for applications across electronics, energy, medicine, and environmental science. However, challenges related to their synthesis, toxicity, and cost must be addressed to fully realize their potential. With ongoing advancements in research, fullerenes are poised to play a transformative role in various cutting-edge technologies, offering numerous opportunities for innovation in the years to come.
References
1. Kroto, H. W., et al. (1985). “C60: Buckminsterfullerene.” Nature, 318(6042), 162-163.
2. Iijima, S. (1991). “Helical microtubules of graphitic carbon.” Nature, 354(6348), 56-58.
3. Lu, X., et al. (2011). “Fullerenes in drug delivery and biomedical applications.” Journal of Materials Chemistry, 21(11), 3667-3675.
4. Parisi, J. L., et al. (2008). “Fullerene-Based Organic Solar Cells: A Review.” Solar Energy Materials and Solar Cells, 92(3), 421-429.
5. Kamath, A., & Sahu, A. K. (2016). “Fullerenes: Synthesis, Applications, and Toxicity.” Frontiers in Nanotechnology, 5(2), 151-158.